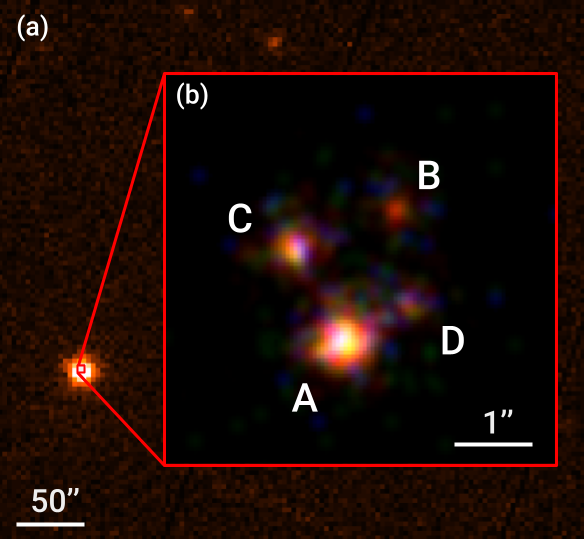
Figure 1: (a) EPIC-pn cleaned image of the first XMM-Newton exposure in the 0.3–10 keV observed-energy band. The red square marks the 5'' region of the Chandra image centered on the quasar and shown in inset (b). (b) Raw Chandra image of Q2237+030 (ObsID 431) binned with a bin size of 0.1'', color-coded based on the observed-energy bands: 0.4–2 keV in red, 2–4.5 keV in green, and 4.5–7 keV in blue. The images are named A, B, C, and D as in Yee (1988). Given the quasar redshift (zQ = 1.695), 100'' separation corresponds to a distance of 8.68 kpc (cosmology values: H0 = 70.0 km s−1 Mpc−1 , Λ0 = 0.73).
- Radio-quiet quasar at z = 1.695
- Lensed in four images (Fig. 1) by nearby galaxy (z = 0.039; Huchra et al. 1985)
- Magnification factor ~ 16 (Schneider et al. 1988, Schmidt et al. 1998; Wertz & Surdej 2014)
- Image time delays ranging from ~2h to ~16h
(Dai et al., 2003; Schmidt et al. 1998; Wertz & Surdej 2014) - Ideal source to study microlensing effects (Dai et al., 2003; Chen et al., 2011, 2012; Mosquera et al. 2013; Guerras et al. 2017)
- Many monitoring campaigns in optical and X-ray band
- 40 X-ray archival observations (as of Sept. 2019)
- 37 Chandra + 3 XMM-Newton exposures
- Spatially resolved in Chandra data
- We refer to Chandra observation using the CXO ObsID
- We refer to Chandra observation using the CXO ObsID
- XMM-Newton exposures provide better counting-statistics spectra, apart from the last observation, which we discard because of high flare contamination
- First XMM-Newton obs.: XMM 2002
- Last XMM-Newton obs.: XMM 2018
- 37 Chandra + 3 XMM-Newton exposures
References:
Chen, B., Dai, X., Kochanek, C. S., et al. 2011, ApJ, 740, L34
Chen, B., Dai, X., Kochanek, C. S., et al. 2012, ApJ, 755, 24
Dai, X., Chartas, G., Agol, E., Bautz, M. W., & Garmire, G. P. 2003, ApJ, 589, 100
Huchra, J., Gorenstein, M., Kent, S., et al. 1985, AJ, 90, 691
Mosquera, A. M., Kochanek, C. S., Chen, B., et al. 2013, ApJ, 769, 53
Schmidt, R., Webster, R. L., & Lewis, G. F. 1998, MNRAS, 295, 488
Schneider, D. P., Turner, E. L., Gunn, J. E., et al. 1988, AJ, 95, 1619
Wertz, O., & Surdej, J. 2014, MNRAS, 442, 428
Yee, H. K. C. 1988, AJ, 95, 1331