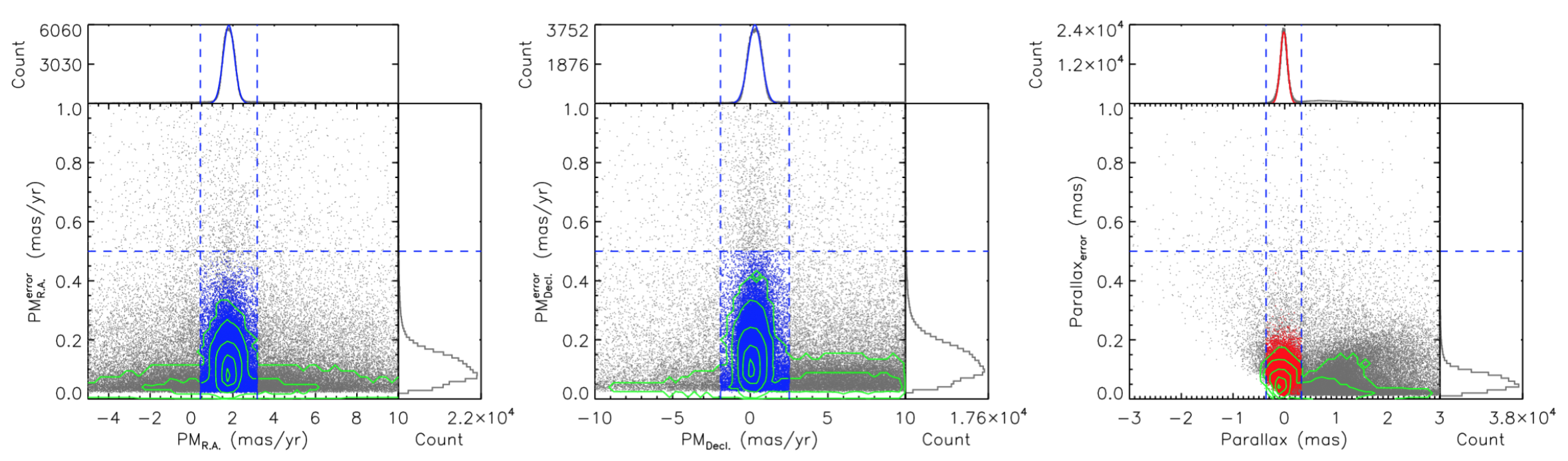
Figure 1. Example of evaluation of the Gaia astrometric solution in the LMC. Membership of the LMC (red) is determined by ±5σ limits (vertical dashed lines) of simultaneous Gaussian profile fittings of PMR.A. (blue), PMDecl. (blue), and parallax (red). For parallax, an additional elliptical constraint is also applied with the 5σ limits of PMR.A. and PMDecl. taken as the primary and secondary radii as also shown in Figure 2, respectively.
We present two clean, magnitude-limited (IRAC1 or WISE1 ≤ 15.0mag) multiwavelength source catalog for the Large and Small Magellanic Cloud (LMC and SMC). The catalogs were essentially built upon a 1′′ crossmatching and a 3′′ deblending between Spitzer Enhanced Imaging Products (SEIP) source list and Gaia DR2 photometric data, with limiting magnitude of IRAC1 or WISE1 ≤ 15.0 mag. We further constrained the proper motions (PMs) and parallaxes from Gaia DR2 to remove the foreground contamination. The membership of the LMC and SMC were defined by simultaneous Gaussian profile fittings in the PMR.A., PMDecl., and the parallax, where an additional elliptical constraint derived from PMR.A. and PMDecl. also was applied to parallax (the 5σ limits of Gaussian profile fitting in PMR.A. and PMDecl. were taken as the primary and secondary radii, respectively) as shown in Figure 1 and 2 taking the LMC as an example. There are 45,466 targets as in the SMC and 197,004 targets in the LMC, respectively. We estimated that about 99.5% of the targets in our catalogs were most likely to be genuine members of the LMC and SMC. Figure 3 illustrates the Gaia color-magnitude diagram (CMD) before (gray) and after (red) applying the astrometric constraints, where the large amount of foreground contamination is swept out. We also indicate the approximate positions of main sequence stars (MSs), blue helium burning stars (BHeBs), and red HeB stars (RHeBs) for the sample of Gaia Collaboration (2018) compared with our sample (the BHeBs and RHeBs were referred to as BSGs and RSGs in our sample). Since our sample was selected based on the infrared criterion (IRAC1 or WISE1 ≤ 15.0 mag), it presumably traces the relatively luminous, cooler evolved stars with a larger mass-loss rate (MLR), such as the BHeBs and RHeBs, than the MSs. However, there will inevitably also be a source of contamination from the MS massive stars at the blue end, which cannot be easily disentangled as shown in the diagram. See more details in Yang et al. (2019, 2021).
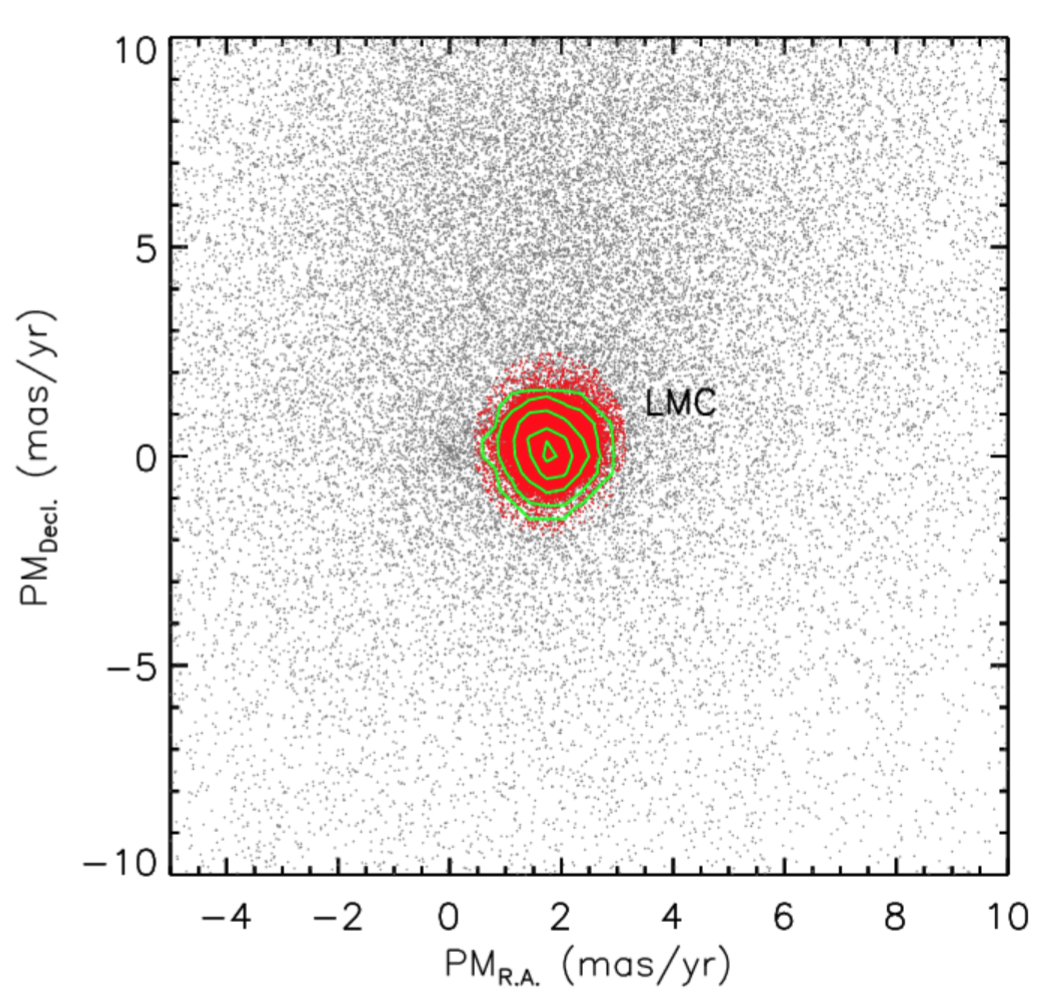
Figure 2. PMR.A. versus PMDecl. diagram, in which the selected LMC members (red) are clearly shown as a cluster.
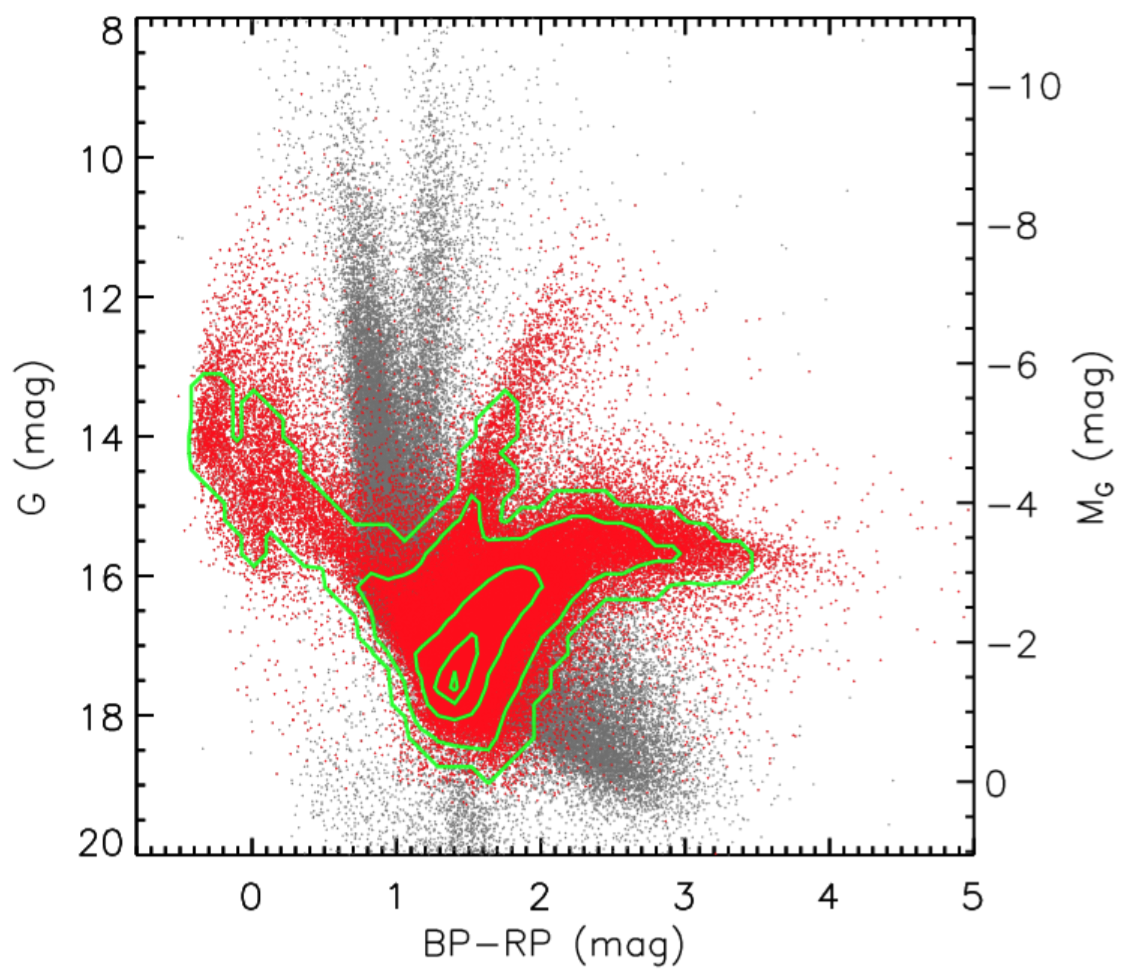
Figure 3. Diagram showing G vs. BP − RP for the Gaia data in the LMC before (gray) and after (red) the astrometric constraints, where the large number of foreground contamination is swept out.
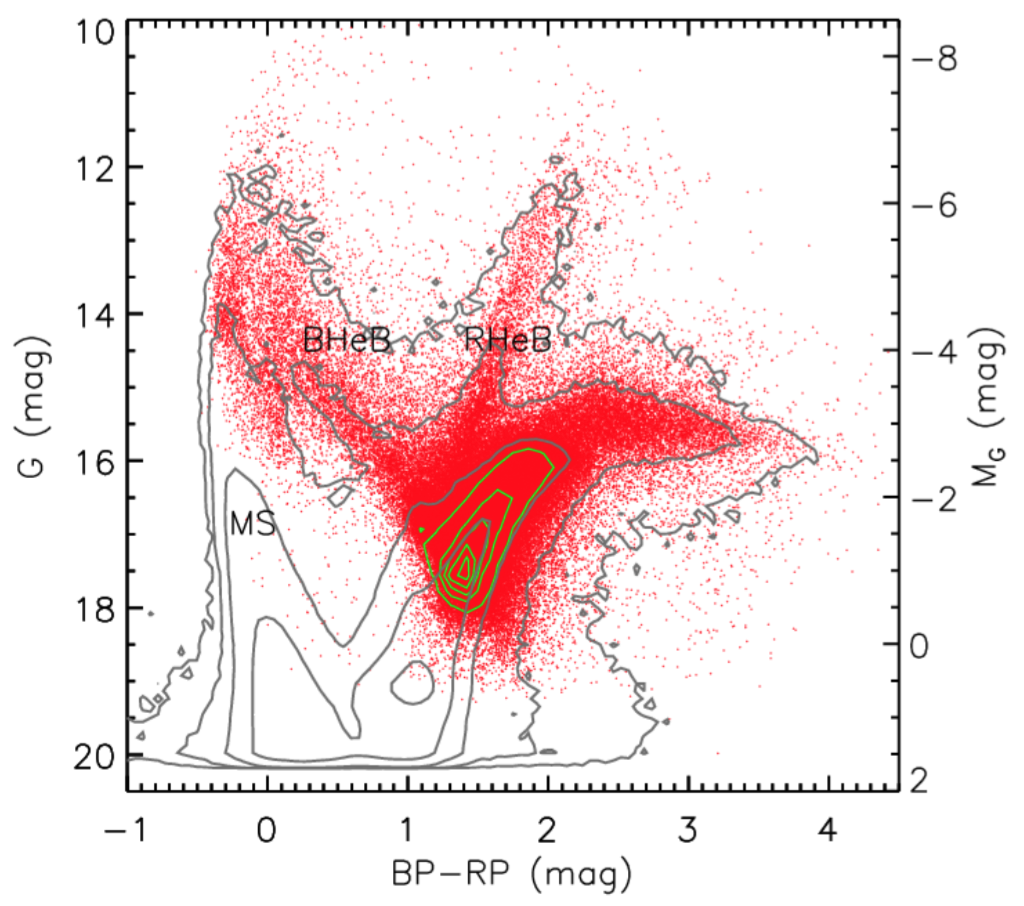
Figure 4. Comparison of Gaia CMDs between our sample and LMC sample from Gaia Collaboration (2018). The approximate positions of MSs, BHeBs, and RHeBs for the sample of Gaia Collaboration (2018) are indicated.