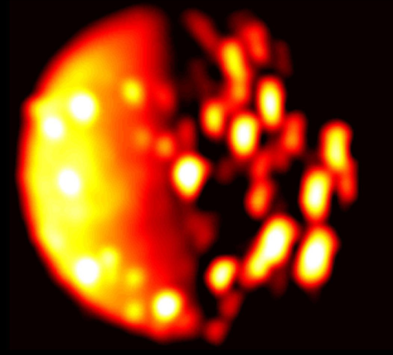
Figure 1: Io in the IR (Credits: NASA/Jet Propulsion Laboratory)
Since more than 200 moons exist in the Solar System, it is expected that they orbit exoplanets as well. Detecting an exomoon could set the next milestone in observations of exoplanetary systems.
Tidal interactions between planets and their satellites can heat a satellite’s interior. The most evident example is Io (Figure 1), which is the most tidally active body in the Solar System. Since tidal dissipation depends on the orbital and physical properties of the system, if tidal heating is vigorous enough in exoplanetary systems, there is a chance that Tidally Heated Exomoons (THEMs; Peters and Turner 2015) are detectable with current instrumentation and/or the JWST in infrared (IR) wavelengths. Recently, Rovira-Navarro et al. (2021) studied the thermal states of THEMs for different orbital configurations and investigated their longevity. As a direct result of tidal heating, spectral signatures of volcanic activity could also be a method of detecting THEMs (Oza et al. 2019).
Tidal dissipation within the satellite is linked to the eccentricity of the orbit (Figure 2). An eccentric orbit causes periodic deformation of the satellite. The latter combined with the viscous nature of the satellite cause tidal dissipation within its interior.
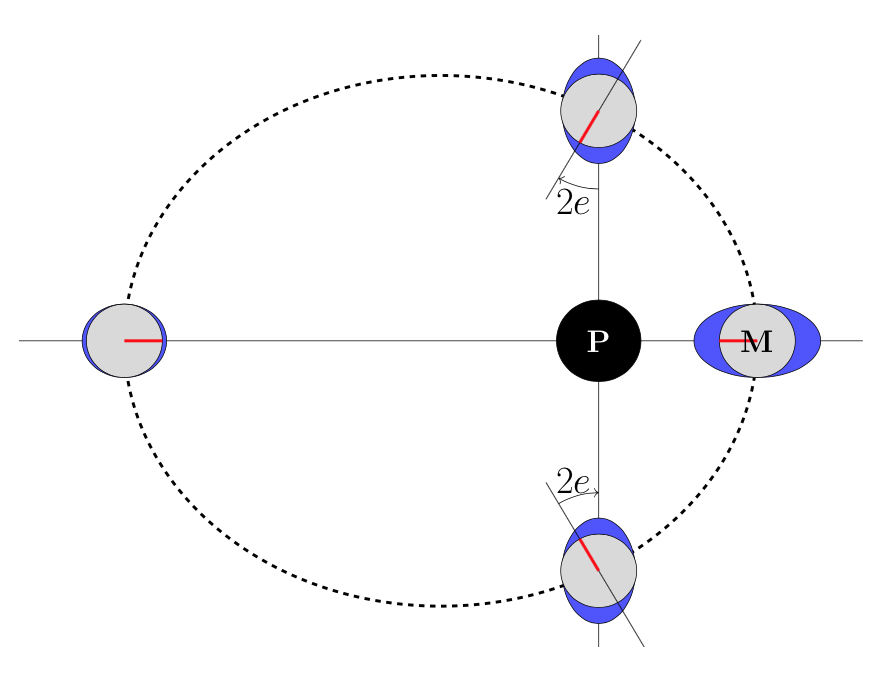
Figure 2: An eccentric orbit causes periodic deformation of the satellite's shape. P represents the planet and M the moon. The tidal bulge that is developed as a result of the interactions between the planet and the moon can be seen in blue.
- Constrain parameter space which would make a THEM observable
- Identify the interior properties which lead to the above values
- Study the diversity of exoplanetary worlds
- Habitable exomoons: detecting a THEM implies the existence of one or more
satellites in MMR retaining the THEM's eccentricity - Place of our Solar System in the Universe
- Insight in planet formation theories