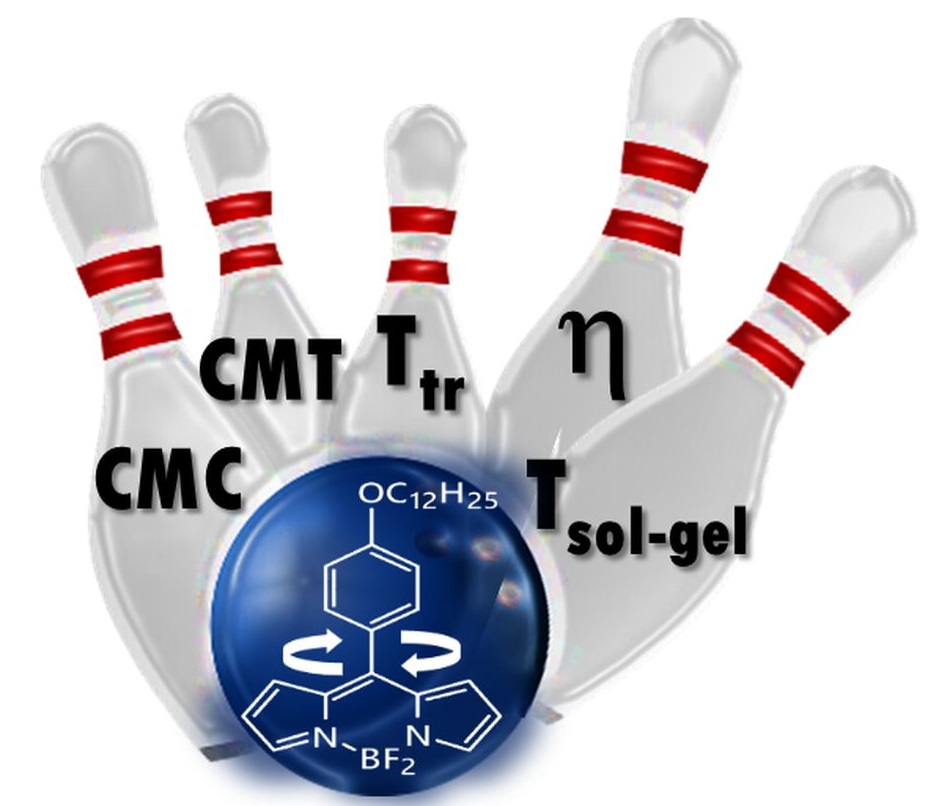
Table caption. Characteristics of self-assembling polymers P123 and F127 determined by fluorescence spectroscopy of molecular rotor BPC12.
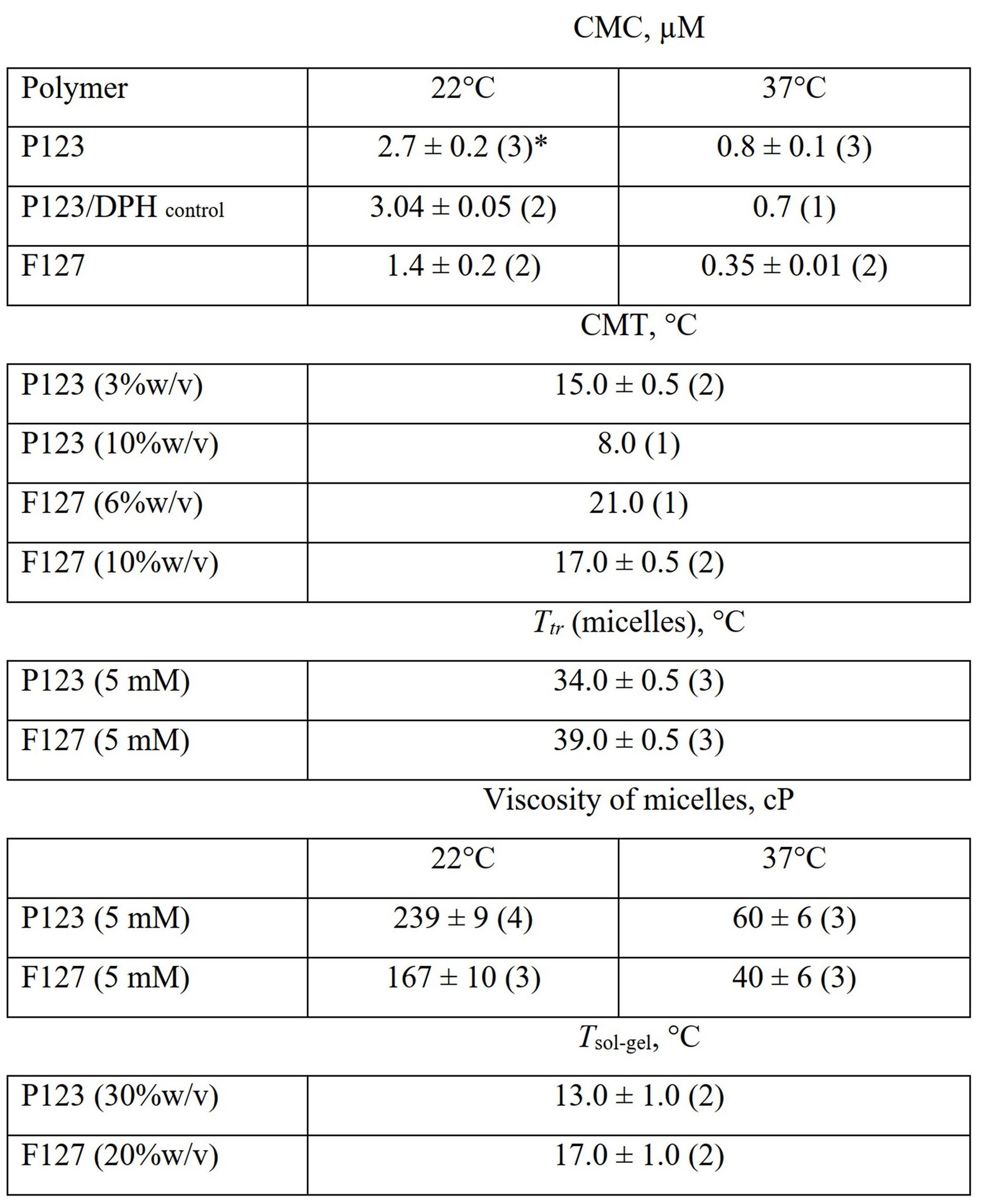
Table caption. Characteristics of self-assembling polymers P123 and F127 determined by fluorescence spectroscopy of molecular rotor BPC12.
CMCs are defined at the intersection points between the two linear regions in the graphs (b) and (c): unimer and micelle regions.
See CMC and CMT values in the summary table in "One sensor - many parameters" section.
Figure caption
(a) Fluorescence spectra of 1.32 µM BPC12 upon titration by increasing concentrations of P123 at 22 °C. Fluorescence intensity of 1.32 µM BPC12 in the presence of different concentrations of the polymers P123 (blue circles) and F127 (red squares) monitored at 517 nm (λex = 490 nm). Plots for the determination of CMC for the both polymers at 22 °C (b) and 37 °C (c).
The temperatures where the fluorescence intensity ceased to increase during the heating cycle or started to decrease upon cooling in graphs (b) and (c) were attributed to critical temperatures of micellization (CMTs).
Figure caption
(a) Fluorescence spectra of BPC12 in 10% solution of F127 corresponding to the plot (b) where arrows show spectral changes upon heating (BPC12 monomer peak at ca. 517 nm and its aggregate – ca. 610 nm). Steady-state fluorescence intensity of BPC12 monitored at 517 nm (λex = 490 nm) as a function of temperature in 10% solution of F127 (b) and 3% solution of P123 (c) in DPBS. Heating cycle – red squares, cooling cycle – black circles. Plots for the determination of CMTs.
Viscosities in the micelles made of both studied polymers P123 and F127 at 22 °C and 37 °C were determined by BPC12 fluorescence lifetime and corresponding calibration curves in graphs (b) and (d).
The core viscosity of F127 micelles was smaller compared to P123 micelles at any temperature suggesting that P123 micelles are more densely packed compared to F127 ones. The viscosities of both F127 and P123 micelles at 37 °C were expectedly lower than at 22 °C (e.g. 60 cP vs. 239 cP for P123)
See viscosity values in the summary table in "One sensor - many parameters" section.
Figure caption
Time-resolved fluorescence decays (a, c) and calibration plots (b, d) of BPC12 in series of glycerol/methanol mixtures of different viscosities.
Clear intersection points between the two linear regions are observed in graphs (b) and (d) at 34 °C for P123 and 39 °C for F127.
The temperatures (Ttr) are independent of the polymer concentration and the polymer/dye ratio and attributed to a phase transition characterizing a change in dynamics of the micellar core - micellar core becomes more fluid upon heating over the Ttr.
See summary table in "One sensor - many parameters" section.
Figure caption
Fluorescence intensity decay curves (a, c) detected at 520 nm (λex = 483 nm) and corresponding calculated lifetimes (b, d) of BPC12 (10 µM) in P123 (5 mM) micelles (upper panel a, b) and F127 (5mM) micelles (lower panel c, d) at different temperatures. The intersection of linear regression fits of the data denotes the Ttr in the micelle core.
Conclusions
Hydrophobic molecular rotor BPC12 can be used for the multiparameter characterization of self-assembling polymers using its strong fluorescence sensitivity to the surrounding environment.
For the first time, molecular rotor’s steady-state fluorescence spectra have been utilized to determine both the critical micelle concentration (CMC) and critical micelle temperature (CMT).
Furthermore, the time-resolved fluorescence spectroscopy of BPC12 allows a novel sensitive, accurate and non-destructive measurment of phase transition in the polymeric micelle core (Ttr) and simultaneously delivers valuable information about hydrophobic micelle core viscosity. The BPC12 molecule is also useful for studying the thermoreversible sol-gel transitions (Tsol-gel).
Thus, the BODIPY molecular rotor is particularly valuable for determining many important features of self-assembling materials with potential for drug delivery applications.
Moreover, the proposed unified approach using fluorescence properties of a single molecule will serve as an attractive, non-destructive alternative to several different techniques conventionally used to determine the same parameters of self-assembling polymeric materials.
Future directions
We strongly believe that the arsenal of parameters measured using the BODIPY sensor can be expanded and the technique presented in this paper can be generalized for other polymeric materials and applications in different fields of polymer-based nanotechnology. We also suggest that the other molecular rotors may show potential for the fluorescence-based investigation of self-assembling materials.
The temperature at the intersection point between two linear regions in graph (b) was attributed to a complete gelation of the solution (Tsol-gel).
The temperatures are 17 °C for 20%w/v F127 and 13 °C for 30%w/v P123.
See summary table in "One sensor - many parameters" section.
Figure caption
(a) Pictures of 20% F127 at 4 °C (sol) and at RT (gel). (b) BPC12 fluorescence lifetime monitored at 520 nm (λex = 483 nm) in 20% F127 in DPBS as a function of temperature. Heating cycle – red squares, cooling cycle – black circles.