Young, pre-main sequence T Tauri and Herbig AeBe stars are known for their characteristic emission line spectra, with narrow and broad profiles and often, absorption features characteristic of winds and infall. The most prominent lines, such as the Balmer Hydrogen series, are very complex and result from combined emission and absorption in many different locations around the star. But young stars have also many metallic lines, including Fe I, Fe II, Ti I, Ti II, among others. These lines are many and may be excited under a large range of energies and densities. Combined with velocity-resolved and time-resolved spectra, emission (and absorption) line tomography can provide valuable information on the properties of the stars at spatial scales that cannot be resolved by other means.
Different methods can be used to analyze the lines. First, velocities and timescales can be converted into spatial scales, providing information on the location at which the variability phenomena are observed (e.g. Dupree et al. 2012, Sicilia-Aguilar et al. 2020). Comparing lines of ionized and neutral species can be used to constrain the densities and temperatures using the Saha equation. The line ratios of lines that originate from the same velocity structure and share an upper level can offer further constraints that are independent of the way the levels are populated (Beristain et al. 1998).
In this poster, we show what we have learn about the innermost structures of several stars with different masses and properties applying this methodology.
Figure on the right: Given a velocity, time resolution can provide spatial resolution. For optical spectra that trace material at the star or very close to it, the timescales of variability are typically days and can be used to map the structures around the forming star, including accretion columns, winds, and the innermost disk.
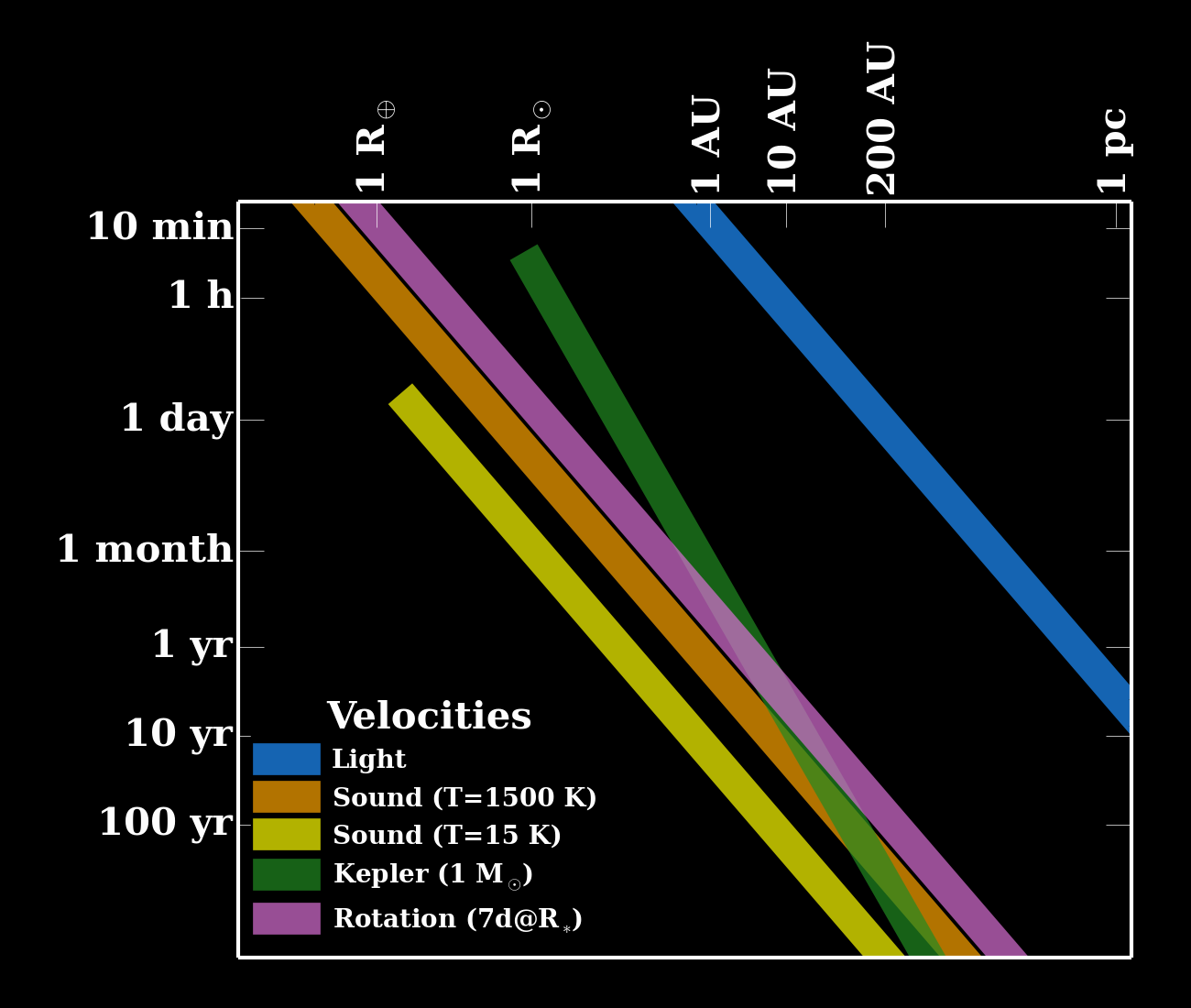